Stem cells, Organs and Organoids on chip
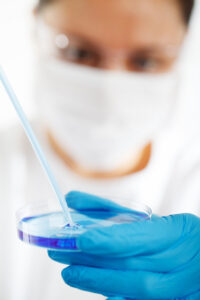
Crédit: Laboratoire Poietis
In vitro means “in the glass”: The reactions take place outside the body. In vitro tests focus primarily on the reaction itself.
In vitro methods are increasingly used not only in the screening stages (techniques for identifying chemical compounds), but also during drug development. The ease with which they can be used, their isolation from any physiological context, which makes it possible to study a toxic mechanism of action, and above all the possibility of using human cells, which means that differences between species can be eliminated, make them an essential tool.
Stem cells
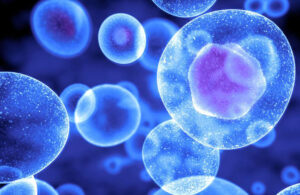
Crédit: Depositphotos
A stem cell is an undifferentiated cell capable of self-renewal, differentiation into other cell types and proliferation in culture.
Stem cells are most often derived from human surgical waste destined for incineration, such as cells found in skin and adipose tissue.
Technological advances have made it possible to programme these adult stem cells. They can give rise to virtually all the different types of cells in the body. They are pluripotent and known as iPSCs (induced pluripotent stem cells). Thanks to their properties, these cells can be used to regenerate or recreate destroyed tissues. Stem cells are used to design organs and organoids on a chip.
Organs and organoids on a chip
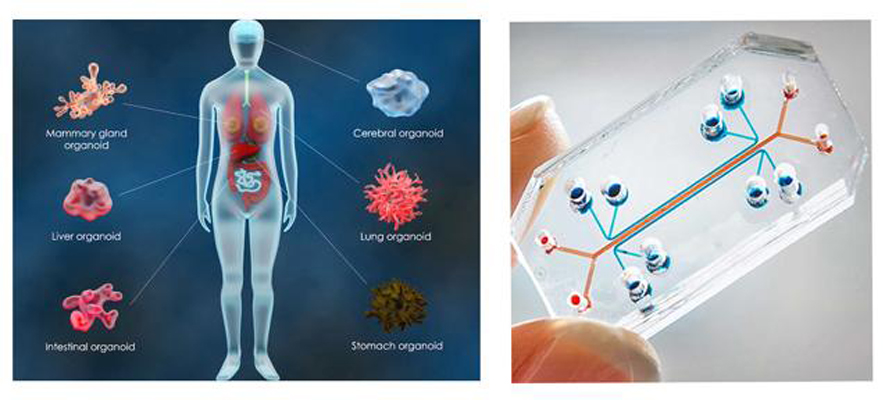
Crédit: iStock
The aim of organoids or organs on a chip is to mimic the architecture and function of organs.
These devices are made up of human cells grown in a microenvironment, in valves punctuated by channels that can be connected using microfluidic technology.
It is designed so that the cells can simulate different functions of the human body.
With this technology, it is therefore possible to study the propagation of a pathogen or the diffusion of a drug in one or all of the organs cultured. It is also possible to simulate the interactions between organs using multi-organ chips.
Organoids are 3D cellular structures that mimic the architecture and function of the entire organ. They are obtained in particular from induced pluripotent stem cells (iPSC).
Clear advantages of in vitro methods over the use of animals
- Accurately study the impact of the chemical reaction
- Offer ease of cultivation and economic advantages for pharmaceutical industry production
- Use human and not animal metabolism for toxicological studies
Some applications
Organoids on a chip to study cancerous tumors
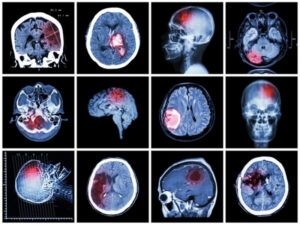
Crédit: Depositphotos
The 3D glimpse project aims to create a tumor on a chip in order to better understand and treat glioblastoma, a very aggressive cancer with an average survival of one year after diagnosis. In this chip, we will seek to represent the tumor micro-environment via the use of different types of human cells organized in 3D in order to form micro-vessels. These will be infused to mimic blood flow in order to study the transport and effectiveness of new nano-drugs.
The liver on a chip to analyze the toxicity of molecules for therapeutic purposes
The MimLiveronChip project is a biomimetic Liver-on-a-chip platform. Developed to recreate the analysis of liver metabolism and xenobiotic toxicity. MimLiveronChip seeks more particularly to explore the effects of the mechanical or biochemical microenvironment influencing the opening of the liver monolayer, in order to be able to generate it or, on the contrary, alter it.
Cell therapy
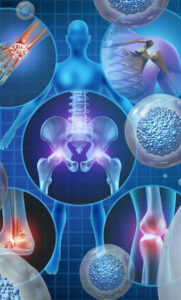
Crédit: Depositphotos
Cell therapy involves using iPSCs (induced pluripotent stem cells) or multipotent cells from the patient or a donor to graft cells to restore the function of a tissue or organ. The aim is to provide lasting treatment for the patient through a single injection of therapeutic cells. Cell therapy can also be used in the manufacture of organoids, for example.